Petrophysical Training
Licenses
|
DEFINITIONS
The "Physics of Petrophysics" is the dominant theme of
this Chapter, which covers most of the underlying principles.
Here we provide the basic physical concepts required to predict
log analysis parameters for gases, liquids, solids, and mixtures.
Much of this
material was covered in high-school and university level courses
in Physics, but the application to rocks containing economic
minerals might have been overlooked. A few initial definitions
are in order.
A
law can be proved, using the most primitive of physical or mathematical
rules, whereas a theory cannot be proved. For example, the Law
of Conservation of Energy can be proved by invoking more primitive
physical laws. The Theory of Relativity cannot yet be proved,
and alternate theories exist, although they are not widely held.
A
good theory explains all the known data, and may even predict
as yet unobserved data, as the Theory of Relativity has done.
A poor theory may still be widely believed, even if it fails to
account for all observed facts. Some believers may discount the
data that does not fit, assuming it is in error, or will predict
that improvements to the theory will allow all data to fit. The
controversy over Creation (now known as Intelligent Design) versus Evolution falls into this category.
An
empirical relationship differs from both a law and a theory. The
empirical relationship is a mathematical "best fit"
between two or more observed sets of data. Many individual data
sets will not follow the empirical relationship well. For example,
it is often true that a larger object weighs more than a smaller
item, but there are many exceptions to that rule.
These
relationships are often termed rules of thumb, and frequently
apply only in limited areas or under very restrictive circumstances.
Some relationships used in log analysis are actually laws, such
as those dealing with the summation of densities in mixtures.
Many, if not most, are empirical relationships, such as the Wyllie
time-average formula, or the Archie formation factor concept.
PARTICLE PHYSICS
For more than a century, we were taught that the elementary
particles of matter were positively charged protons, neutral
neutrons, and negatively charged electrons. All matter in the
universe was thought to be made up of stable, and some unstable,
combinations of these three particles, forming larger particles
called atoms. The particles are held together to form elements
by forces of attraction between the basic particles.
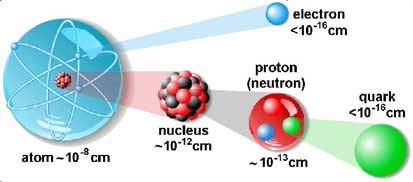
Basic particles are pretty small.
For comparison, the Universe is 10^28, the
Milky Way is 10^23, the
Solar
System is 10^15, and Earth is 10^9 cm in diameter
More
recently, nuclear physicists have proposed the "Standard Model", showing that these
so-called "basic particles" are actually made of even smaller
elementary
particles called, naturally enough, sub-atomic particles. There are two
types of subatomic particles: elementary particles, which are not
made of other particles, and composite particles.
Some scientists have postulated
that these elementary particles are composed of even more basic
particles called preons (not to be confused with prions, a type of
protein). No evidence exists to support this conjecture.
The
elementary particles of the Standard Model include:
* Six "flavours" of Quarks: up, down, bottom, top, strange,
and charm
* Six types of Leptons: electron, electron neutrino, muon,
muon neutrino, tau, tau neutrino
* Thirteen gauge Bosons (force carriers): the graviton of
gravity, the photon of electromagnetism,
the three W+,
W-- and Z bosons of the weak force, and the eight gluons of
the strong force.
Composite
particles include all hadrons, subdivided into two categories: Baryons
and Mesons.
Composite subatomic particles (such as protons or atomic nuclei) are
bound states of two or more elementary particles. For example, a
proton is made of two up quarks and one down quark, while the atomic
nucleus of helium-4 is composed of two protons and two neutrons.
Hadrons
are any
strongly interacting composite subatomic particle.
All hadrons are composed of quarks.
Baryons
are strongly interacting fermions such as neutrons
and protons, made up of three quarks.
Mesons
are strongly interacting bosons consisting of a
quark and an antiquark.
QUARKS
Abbrev Elec Charge Mass
Up
u +2/3
2 MeV Stable
Down d
-1/3
5 MeV Stable
Two Up quarks and 1 Down quark make a Proton with net charge of +1.
Two Down quarks and 1 Up quark make a Neutron with net charge of
0.
Charm C
+2/3
1.25 GeV Unstable
Strange S
- 1/3
95 MeV Unstable
Top
t
+2/3
171 GeV Unstable
Bottom b
-1/3
4.2 GeV Unstable
The unstable quarks make up short-lived particles, seen only in very
high energy physics labs and cosmic rays.
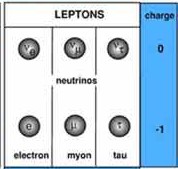
LEPTONS
Abbrev Elec Charge
Mass
Electron e
-1
0.511 MeV Stable
Muon u
-1
105 MeV Unstable
Tau
T
-1
1.78 GeV Unstable e .
There are three
Neutrinos
corresponding to each of the three
leptons. Neutrinos have no charge and rarely interact with ordinary
matter.
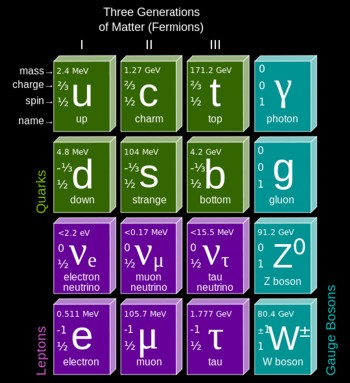
Antiparticles equivalents to the quarks and leptons
exist, such as positrons,
antiprotons, or antineutrons, having the same mass, average
lifetime, spin, magnitude of magnetic moment, and magnitude of
electric charge as the particle to which they correspond, but having
the opposite sign of electric charge, opposite intrinsic parity, and
opposite direction of magnetic moment. They exist today
only in high energy particle accelerators but were abundant, in
theory, in the early moments of the Big Bang .
Fermions
comprise all particles with spin of 1/2. These are the 6
quarks, 6 anti-quarks, 6 leptons, and 6 anti-leptons.
Period Table of Quarks, Leptons, and Bosons 
Photons
carry electoomagnetic energy, such as light, radio waves, and gamma
rays.
Gluons
come in eight different species. They carry the strong force that
binds quarks into other particles.
Bosons carry
the forces that act to bind or attract particles. The most obvious boson is the
photon, the carrier of electromagnetic radiation (eg: light, radio,
television, gamma rays, X-rays). Photons can have an effect over
huge distances. Photons can behave as particles or waves, leading to
a duality that underlies much of quantum physics.
The Z
boson, W-
boson, and W+ boson operate over very tiny inter-atomic distances
(10^-18 meters), carrying the weak force.
The Higgs boson (graviton), postulated to carry the force of
gravity, may have been discovered in 2012 at the Large Hadron
Collider at CERN in Geneva. If it exists, theory predicts that it
has a mass greater than 125 Gev.
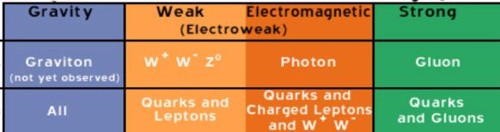
Particles carry the weak and
strong forces
DARK ENERGY |
Dark Energy is a
hypothetical form of energy that permeates all of space
and tends to increase the rate of expansion of the
universe. It is the most popular way to explain
observations that the universe appears to be expanding
at an accelerating rate. In the standard model of
cosmology, dark energy currently accounts for 74% of the
total mass-energy of the universe.
|
Dark Matter involves heavy but virtually
undetectable particles called neutralinos (not to be confused with
neutrinos). Neutralinos need a mass of 100 to 1000 times that of a
proton. They are also called weakly interacting massive particles (WIMPs)
and have not been detected directly.
Another model proposes a different
particle, the axion, that is one trillionth the mass of an electron.
It will take quite a few of them to make up the missing mass.
Dark matter is weakly or non-interacting, so it is
called nonbaryonic matter. It's composition is as
yet unknown.
Atoms
consists of at least one proton and one electron (hydrogen). The
nucleus of all other atoms consists of protons and neutrons,
surrounded by electrons.
Elements
are made of one or more atoms with the same number of protons. An
element cannot be broken into smaller elements by ordinary chemical
processes. Helium, oxygen, sodium or chlorine are elements. There
are 117 elements known to date, the heaviest being unstable and very
short-lived. Unstable elements are said to be radioactive, decaying
in time to some lighter, more stable element.
Atomic Number (Z)
represents the number of protons in an atom and
uniquely identifies a chemical element. The number
of electrons surrounding the nucleus equals the
number of protons.
Atomic Weight (A),
or
Mass Number, is the number of protons plus
neutrons in the nucleus of an atom.
Isotopes of an element have the same number of protons and
electrons (same Atomic Number Z), but different
numbers of neutrons. Some isotopes are stable, some
are radioactive. About 339 isotopes occur naturally
on Earth, of which about 79% are stable. Counting
the radioactive isotopes not found in nature that
have been created artificially, more than 3100 are
currently known.
Unstable isotopes decay to more stable forms, some
of which may be unstable and decay further. The
decay process gives off radiation. The time it takes
for the unstable material to decay to one half its
original mass is called the half life.
For example, 93% of potassium atoms have 19 protons
with 20 neutron and are stable, giving an atomic
number of 19 and an atomic weight of 39. One
particular isotope
has 21 neutrons, giving an atomic weight of 40. It
is unstable and comprises only 0.012% of all
Potassium atoms. Other isotopes, some stable, some
not, make up the remaining 7% of the atoms.
RADIOACTIVITY
The common forms of radioactive decay are by
emitting Alpha, Beta, or Gamma particles (or
rays), There are other more complicated forms of
radioactivity not described here, for example
various methods of inducing neutron emissions for
use in medicine or well logging.
Atoms in a
radioactive substance decay in a random
fashion but at a characteristic rate. The
length of time this takes, the number of
steps required, and the kinds of radiation
released at each step are well known from
laboratory measurements and quantum theory
calculations.
Half-Life
is the time taken for half of the atoms of a
radioactive substance to decay. Half-lives
can range from less than a millionth of a
second to millions of years depending on the
element concerned. After one half-life the
level of radioactivity of a substance is
halved, after two half-lives it is reduced
to one quarter, after three half-lives to
one-eighth and so on.
Alpha Decay
is a type of radioactive decay in which two protons
and two neutrons are emitted. They are bound
together into a particle identical to a helium
nucleus. The original atom transforms into an atom
with a mass number 4 less and atomic number 2 less
than the original atom. A common example is the
decay of Uranium-238 into Thorium-234. Two electrons
are also stripped from the original atom.
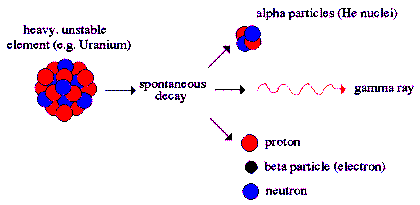
Alpha radiation
produces a helium atom and one or more gamma rays
There
is a difference in mass between the original nucleus
and the sum of the mass of the
particle and
resulting nucleus. This lost mass is converted into
energy using the formula E = mc2;
the energy would equal the kinetic energy of the
particle and the
recoil energy of the resulting nucleus.
Excess energy,
if any, is released as one
or more gamma rays. For example, some Radium decays
to Radon give gamma rays, some do not. Some alpha events never produce gamma rays.
Alpha particles cannot travel far
and are not particularly dangerous unless ingested.
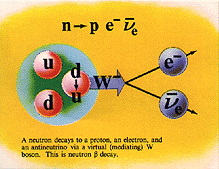
Beta Emission
is a process in which an electron and an
antineutrino (or a positron and a neutrino)
are emitted. In the case of electron emission, a
neutron is converted to a proton and is referred to
as "beta-minus", and the atomic number is
increased by 1. No gamma rays are emitted .
In the case of a positron
emission, a proton is converted into a neutron and is
called "beta plus". The positron is
quickly annialated by a nearby electron and two
gamma rays are emitted. the atomic number is
decreased by 1.
For Potasium-40, beta-minus
results in Calcium-40 and Beta-plus results in Argon-40.
Both daughter products are stable. Gamma rays are
produced in Beta-plus but not Beta-minus events.
A
third form of Beta decay, called Inverse Beta, or
Electron Capture, converts a proton to a neutron by
capturing an inner shell electron, and emitting the
excess energy as a low energy gamma ray (X-ray). For
Potassium-40, this mode of decay also results in
stable Argon-40. Since K-40 has a half-life of more
than a billion years, gamma rays are constantly
being produced and can be detected by conventional
instrumentation.
Some elements decay to a stable form through a
series of alpha and beta emissions, the longest
chain being that for uranium, shown below. Note that
each Beta-minus decay increases the atomic number by
1 and each Alpha decay decreases it by 2.
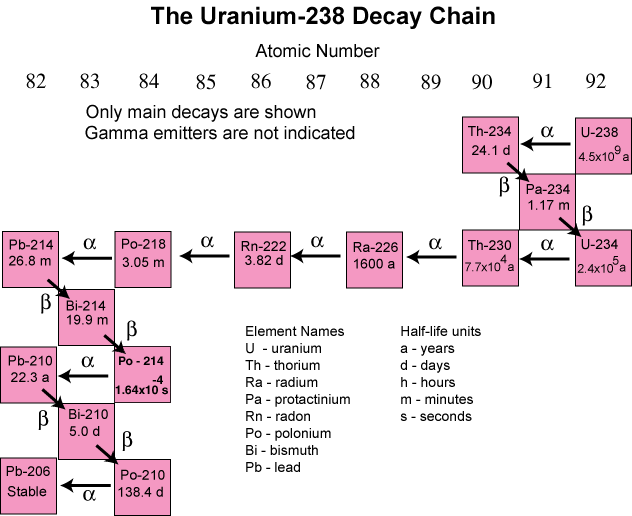
Decay chain for Uranium-238 to stable Lead-206
Gamma Rays
are
high energy photons,
a form of
electromagnetic
radiation, produced
by sub-atomic
particle
interactions, such
as electron-positron
annihilation or
radioactive decay.
Gamma rays are
generally
characterized as
having the highest
frequency and
energy, and also the
shortest wavelength
(below about 10 picometers).
Hard X-rays overlap the range of
long-wavelength (lower energy) gamma
rays, however the distinction
between the two terms depends on the
source of the radiation, not its
wavelength; X-ray photons are
generated by energetic electron
processes, gamma rays by transitions
within atomic nuclei.
Due to their high energy content,
X-rays and gamma rays can cause serious damage
when absorbed by living cells.
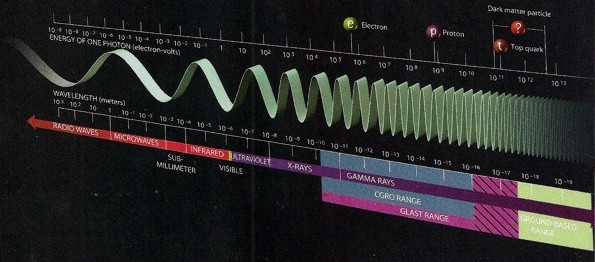
Energy and frequency of major
sub-atomic particles (click image for larger
view)
In well logging, the natural gamma
radiation from rocks is used to to assist in assessing mineralogy of
the rocks. Another well logging tool emits neutrons, either from a
chemical or accelerator source in the logging tool, to help assess
mineralogy and porosity. Instruments that emit gamma rays are also
used for similar purposes.
Photo-electric Effect
occurs when a low energy photon, such as light,
strikes an electron in an atom. The photon is absorbed and an
electron is ejected from the atom, provided the photon has
sufficient energy. The phenomenon was described by Hertz and others in the late 1800's
and by Einstein in 1905. Einstein's experiments demonstrated
the quantum nature of photons.
Compton scattering was described in 1923. High energy photons, such as gamma
rays, react somewhat differently rhan low energy photons. In this case, the photon kicks out
one electron, but the photon continues moving on at a lower energy. The fact that the photon loses energy demonstrates its
wave-like characteristics.
The photoelectric
effect takes place with photons with energies from
about a few electronvolts to over 1
MeV. At higher photon energies comparable to the
electron rest energy of 511 keV, Compton scattering
takes place, and above twice this (1.022 MeV) pair
production takes place. However, like all
radioactive events, these reactions are statistical
in nature, so there is no distinct energy boundary
between the three processes.
In well logging, Compton
scattering of gamma rays is used to measure the electron density of
rocks. This is transformed into density in grams/cc. At the same
time, the energy of the scattered gamma rays is measured and
transformed into a value called the Photo Electric Effect (PEF), This is a
little confusing since the measurement is made from scattered gamma
rays, and not from a direct measure of the ejected electrons, as was done
in the 1905 Einstein experiment.
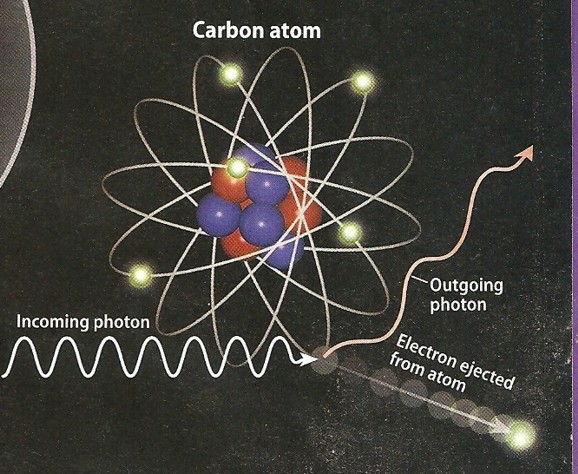
Photo electric effect (left) and Compton scattering (right)
show low energy and higher energy reactions to impinging photons.
ANIMATIONS
FROM: ThinkQuest.com
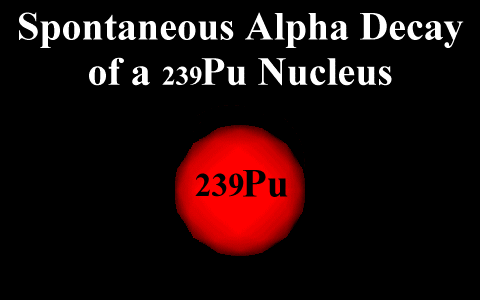
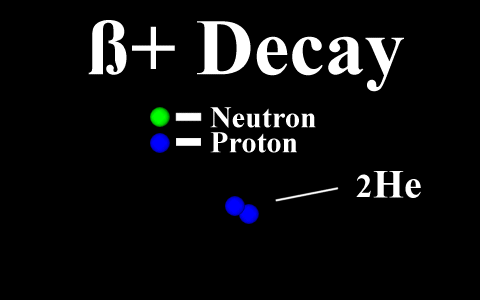
|
|