HYDROGEN BASICS
Hydrogen is the smallest and lightest element. At standard
conditions hydrogen is a diatomic gas (H2). It is
colorless, odourless, tasteless, non-toxic, and highly combustible,
creating water (H2O) when burned. Hydrogen can be
separated from water by electrolysis and from methane by pyrolysis
or steam reforming.
There is one known example, in Mali, of naturally occurring hydrogen
in a geologic setting, It is a small accumulation but is
revolutionizing geological thought on possible sources of natural
hydrogen. Dozens of hydrogen seeps are known around the World – some
of these may prove to be more than just curiosities.
Most of the hydrogen on Earth exists in water and organic compounds,
and in hydrides inside the Earth.
Known occurrences of natural hydrogen are rare, partly because we
haven’t looked very carefully, due to preconceived opinions that are
now known to be incorrect.
Major uses of hydrogen are making ammonia, upgrading bitumen and
heavy oil, and removal of sulphur from liquid petroleum,
industrial and agricultural chemicals, as well as food
processing.
A new era of hydrogen powered aircraft, railway locomotives, ships,
and ground transport is being led by
innovative entrepreneurs and both large and small business ventures.
So far, very tiny steps forward on a very, very long road to the
“Hydrogen Economy” – think year 2050 or beyond. The virtue of such a
fuel is that the exhaust is water (and maybe some NOx), instead of
CO2, which contributes to climate change. There
are many unresolved technical and practical issues, not the least of
which is what to do with all that water in cold weather. Hydrogen
has the potential to assist the global race for decarbonization.
Stay tuned!
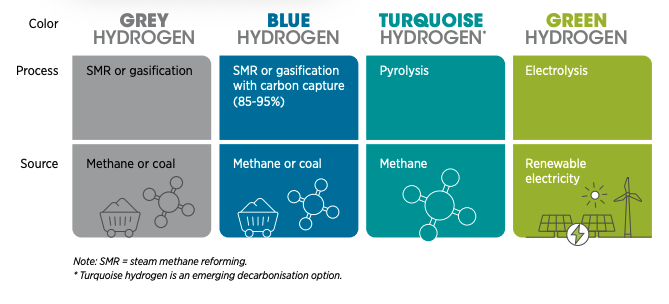
The Colours of Hydrogen: green if produced from 100% renewables;
black, brown, or grey if coal or methane is used; blue if CCS is
added, gold or white if source is naturally occurring. (Image from
World Economic Forum, from 2022 talk by Emanuele Taibi)
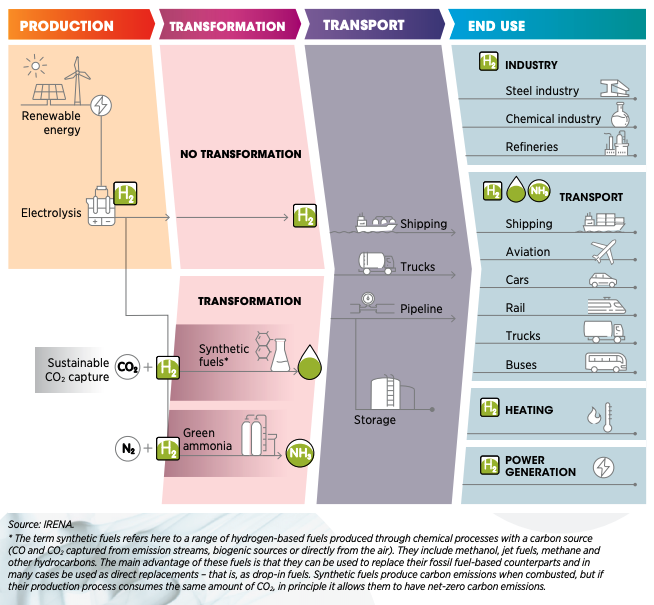
The Green Hydrogen Transition (Image courtesy International
Renewable Energy Agency)
To produce enough Green Hydrogen to displace fossil fuels, we need
to increase renewable electrical energy output by a factor of 1000,
probably much more. And drill and complete unknown thousands of deep
water wells, plus build a desalinization plant for each electrolysis
plant. Why? Because most of the fresh water needed for electrolysis
is already allocated for human and agricultural use.
It might be better to electrify transport and use heat pumps for
HVAC and avoid the H2 middleman. This leaves about
40% of current carbon emissions to be fixed – the carbon-heavy
industrial heartland to decarbonize with Green Hydrogen. As hydrogen
technology improves, the timing might just work out for all those
year 2050 targets that governments have made.
Petrophysical analysis in Hydrogen bearing rocks
Petrophysical
analysis in a hydrogen accumulation is truly difficult,
unconventional, and still open to improvement.
Natural hydrogen gas accumulations do not behave on well logs in the
same way as methane gas reservoirs. Hydrogen gas does not exhibit
high resistivity like a methane gas zone. This phenomenon is not
fully understood but may be related to ionization of H2 in the water
in the rock.
When a hydrogen atom dissolves in aqueous solution, it ionizes into
H+ (a proton) and H- (an electron). Protons cannot live in isolation
and immediately hook up to a water molecule, creating the ion H3O+,
called hydronium. As the protons are used up, more hydrogen can be
dissolved and more hydronium is created. H3O+ ions are conductive,
similar to other Group 1 elements, such as sodium (Na+) and
potassium (K+). As a result, conventional water saturation
equations make hydrogen zones look like water zones.
The electrons released during ionization form a telluric
currennt, visible as large negative excursions on the SP
log.
Hydrogen does not produce density neutron crossover (gas effect).
Instead, H2 shows up as if the zone were shale or heavy minerals –
high neutron porosity from higher hydrogen index (HI) with slightly
high density porosity, giving density neutron separation instead of
crossover. The gamma ray can usually distinguish if it is shale or
non-shale rock.
When methane co-exists with hydrogen, open hole logs behave more
like they would in conventional gas zones.
H2 UPDATE JULY 2023
The neutron log responds to all hydrogen in the rock:
1. water in the effective porosity of the reservoir rock,
2. water bound to clay, shale, or gypsum in the reservoir rock,
3. hydrogen diffused into the rock matrix,
4. hydrogen adsorbed onto the various rock surfaces,
5. hydrogen bound chemically to form hydrides
6. hydrogen dissolved in the water as hydronium ions H3O+,
7. and (maybe) hydrogen in the form of free gas in the effective
porosity.
Items 1 and 2 represent the state of a reservoir before any H2 is
formed or migrates into the reservoir.
Items 3 through 6 must saturate their host to the full extent
possible, limited by the temperature and pressure, and
characteristics of each of the physical or chemical processes.
Only then can free H2 gas survive as an accumulation underground.
When producing from a natural or storage reservoir, hydrogen that
diffused or formed hydrides is permanently lost. Some adsorbed gas
and some free gas will be available for production as the reservoir
pressure is reduced. Some natural H2 plays may offer only adsorbed
gas with no free gas in the porosity.
Detailed sample descriptions are critical in determining the actual
mineralogy, since standard 2- and 3-mineral models are unlikely to
behave well in an H2 zone. Multi-mineral models might work, but the
petrophysical properties of H3O+ are as yet unknown.
Hydrogen can
be seen on the mud log C1 gas curve and as a temperature log anomaly
which shows the hydrogen accumulation as a gas cooling effect
compared to geothermal trends.
Fracture intensity, formation dip, and depositional environment can
be determined from resistivity image logs.
Reservoir seal integrity is critical due to the small size of the H2
molecule, which can leak through almost any trap that would contain
CH4, CO2, N2, or He. The best possible seals are lava flows and
evaporites. You still need a stratigraphic or structural trap,
otherwise the H2 will “just keep on a-movin’ ”.
New and
evolving technology may help. One possibility is the fast neutron
cross section measurement (FNSX). Low density CH4 and CO2 have very
low FNXS values, as well as low SIGMA values, compared to water,
heavier hydrocarbons, and rock minerals. A direct calculation of gas
saturation is be possible in these cases. The FNXS and SIGMA values
for H2 and H3O+ in an accumulation setting are currently unknown, so
we will wait and see what develops.
Elemental yields from a slim hole induced gamma ray spectroscopy log
(eg Schlumberger Pulsar log) might resolve the presence of hydrogen
or hydronium-ions. It should be possible to tune the element to
mineral transform to include H2 and H3O+ in the allowed “mineral”
list.
It has also been observed in ROKE Quad Neutron logging that the
borehole resistivity measurement correlates with the Mudlog H2
signal. One pass with this slim hole logging tool is all that is
needed to identify a hydrogen gas accumulation.
NATURAL HYDROGEN EXAMPLE --
MALI
Conventional literature says that hydrogen gas does not occur
naturally in convenient accumulations like oil and natural gas
reservoirs, because the small molecules could escape too easily.
This is not the case, as a hydrogen accumulation is being exploited
in the region of Bourakebougou in Mali, producing electricity for
the local village.
Tested in 2012 from a capped wellbore machine-drilled for water in
1987, natural hydrogen flowed from below the plastic casing cemented
to the bottom of the wellbore. Analysis of this shallow GazBougou1
discovery well confirmed H2 gas at a concentration
of 98% purity, with traces of methane, and nitrogen. This is the
purest naturally occurring hydrogen ever discovered.
Further exploratory wells were drilled long after the first two
stratigraphic holes F1 and F2 had their cores studied to begin
defining the regional geological model for H2.
Mali’s natural hydrogen is gathered in
5 rock layers, trapped by subsurface lava flows. Deep, medium, and
shallow sources are believed to be at work to periodically refresh
the accumulations of geologic hydrogen. There are at least 7
possible mechanisms for the generation of hydrogen discussed in the
reference paper. There are many challenges in defining hydrogen
system logic, so there are still many unknowns.
This is where petrophysics comes to the rescue. Take a peek under
the rug and see what might be waiting below all those volcanics you
drilled through over the last 70 years. No, it won’t be easy, as you
probably will need faults to the basement and fractures, where well
logs can help there too.
It’s time for a paradigm shift for hydrogen!
Some scientists believe geologic hydrogen gas produced in Mali will
continue for thousands of years, sustainably decarbonising the local
community (even though they did not have much of a carbon footprint
to begin with). This is highly speculative as it may have taken
millions of years for the gas to accumulate.
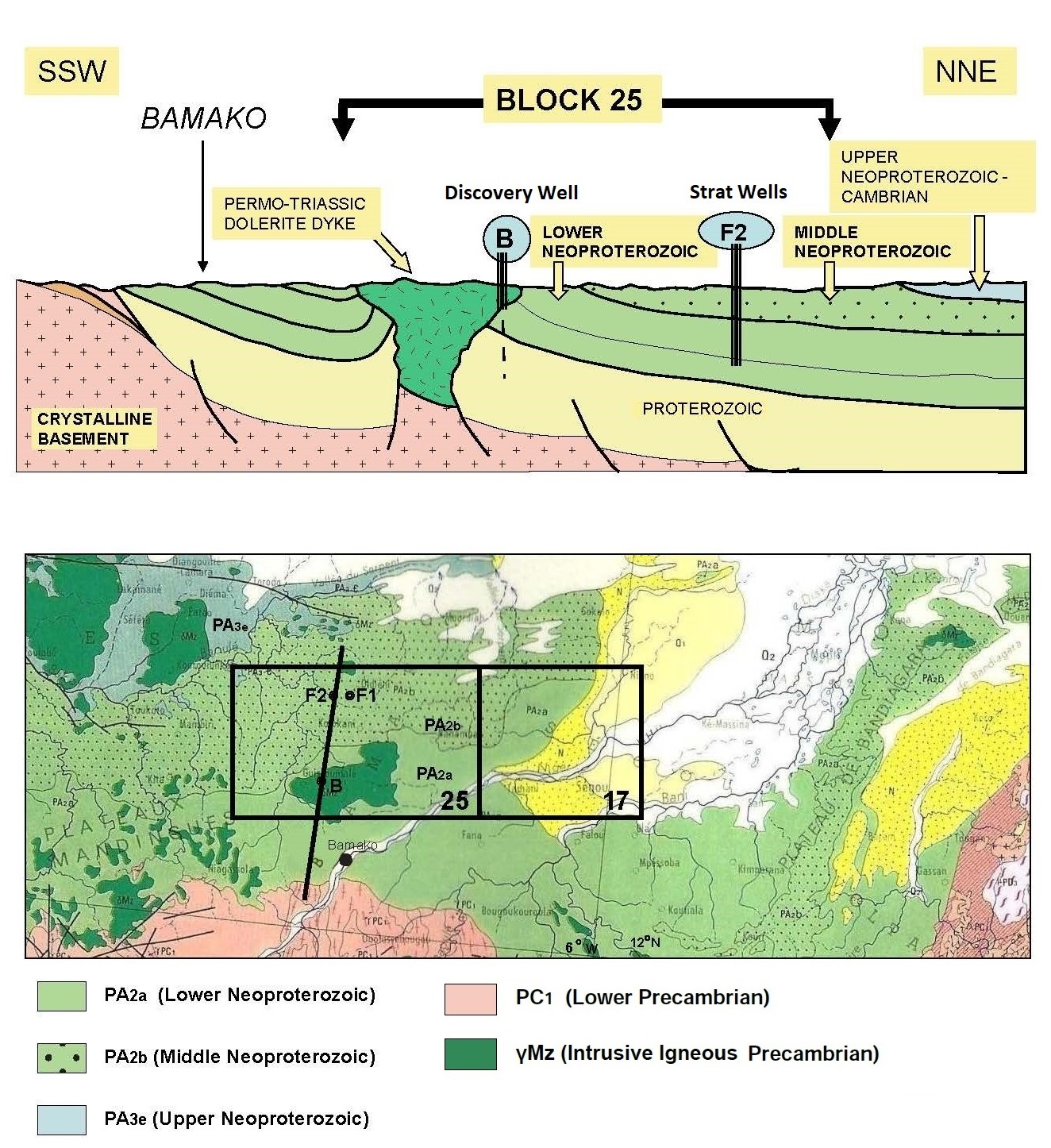
Stratigraphic sequence of Mali natural hydrogen discovery (Ref 1)
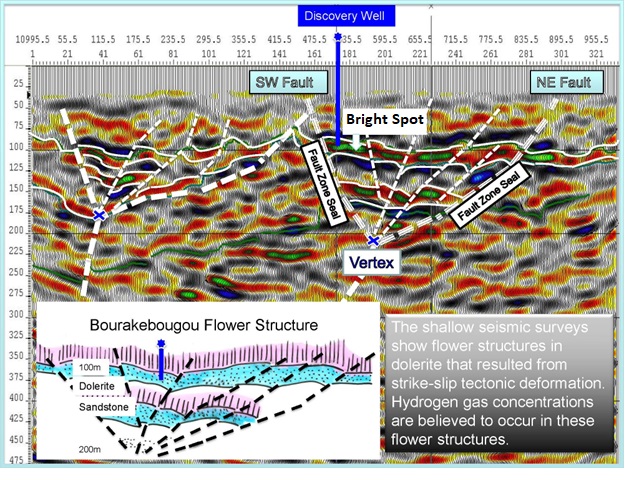
Seismic cross-section of Mali natural hydrogen discovery showing
“flower structure” (Ref 1)
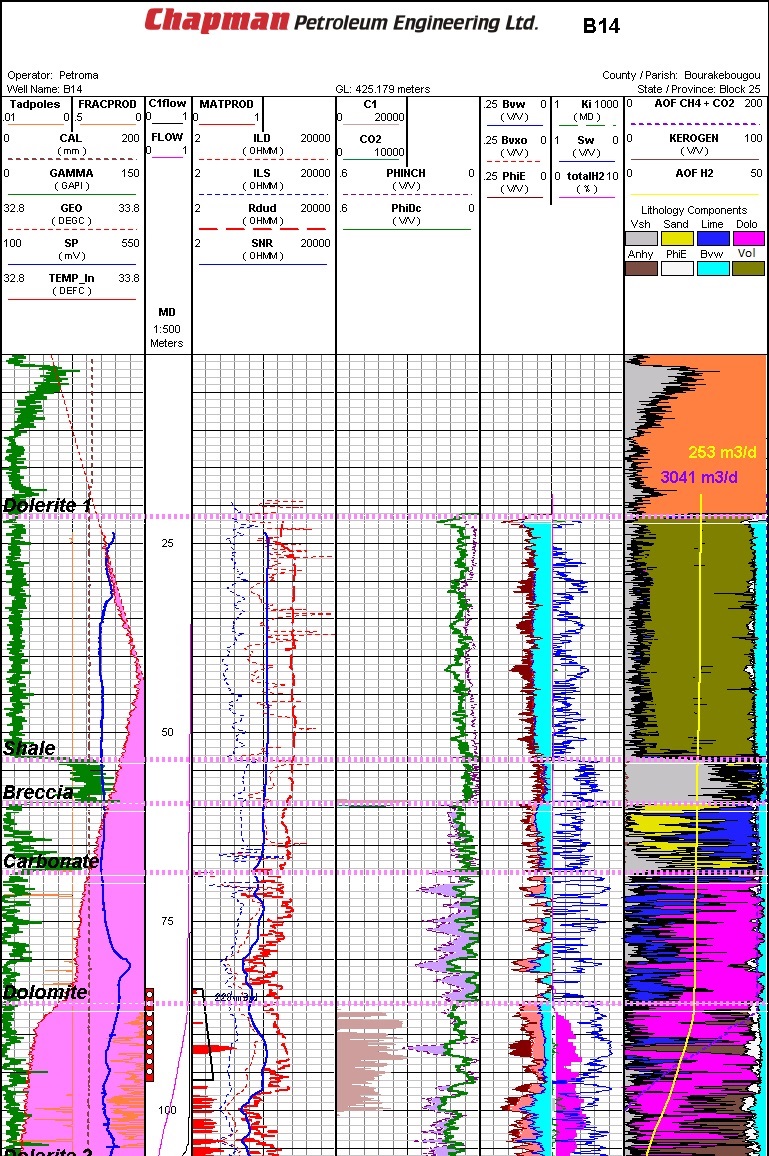
Well log from typical Mali natural hydrogen discovery area (Ref 1)
Hydrogen from Radiolysis in Radioactive Rocks
The three long-lasting naturally occurring chemical elements in the mantle
and crust of the earth, Thorium-232, Uranium-238, and Potassium-40, decay
and give off radioactive particles. Half of the earth’s internal heat comes from
this radioactive decay.
- An isotope of Th-232 gives off alpha and beta particles with half-lives of 14 billion years.
- An isotope of Ur-238 gives off alpha particles with a half-life of 4.5 billion years.
- An isotope of K-40 gives off beta and gamma particles with half-lives of 1.25 billion years.
Beta particles have short interactive paths, and alpha particles have longer particle paths.
Gamma particles only interact once in a photoelectric capture.
When these radioactive particles strike water and surrounding rock matrix, the interaction
causes a removal of an electron from absorbing species all along the particle’s path as the
particle’s energy is depleted. This makes ions of all the absorbing species which quickly
become chemically reactive and subsequently interact with other nearby substances.
Some of the resulting chemicals from this subsequent reaction of radiolysis produce
molecular hydrogen, hydrogen peroxide, oxygen, and helium. It has been estimated that the
earth’s crustal and oceanic radiolytic hydrogen is produced at a rate of 0.47 x 1011 Moles/year.
These facts would indicate that hydrogen should be found in its native states in
radioactive rocks of any kind whether igneous or sedimentary. This includes, for example,
the Canadian shield, any igneous or metamorphic rocks. As of 2024 geological exploration of the
Canadian shield in Quebec is being undertaken to see if water and possibly hydrogen can actually
be located. This will use standard exploration techniques such as stratigraphic borehole drilling,
cores and sample collection. They may be logged with conventional or slimhole logging tools,
for example: gamma ray, sonic, density, neutron, and modern versions of induced gamma ray spectroscopy logs.
Water, if found, would be collected and sampled for its chemistry and hydrogen in solution.
Because we know of several hundred hydrogen seeps in the world it is possible this form of
exploration will find source for many more seeps of this kind. Over the next few years
exploration for this source of natural hydrogen may prove that hydrogen can be produce
at commercial quantities.
Potential
Organic SourceS of Natural Hydrogen
There are two possible geological processes that could
produce natural hydrogen from organic matter (petroleum).
Both involve a form of decomposition or “cracling” of
existing hydrocarbons at depth.
GRAPHITIZATION
The first is graphitization of pyrobitum, in which the final
product is pure graphite in the pore space. I actually
analyzed a 100+ foot graphite bearing limestone on Melville
Island in the Canadian Arctic back in the 1970s – drill
cuttings and an ohm-meter proved the presence of conductive
graphite. To get pure carbon, we need to lose the hydrogen
in a process called dehydrogenation or graphitization..
Evolution of hydrocarbon, showing formation of graphite at bottom
right. The hydrogen (not
shown) is free to move or to become bonded chemically or
physically in the rock.
The precursor for graphitization is pyrobitumen (petroleum coke).
Under high pressure and temperature (pyrolysis),
the coke
passes through a fluid stage (carbonization). This fluidity
facilitates the molecular mobility of the aromatic molecules,
resulting in intermolecular dehydrogenative polymerization reactions
to create aromatic, lamellar (disc-like) molecules. These
“associate” to create a new liquid crystal phase (meso-phase). A
fluid phase is the dominant requirement for production of
graphitizable carbons. Hydrogen is expelled during this
reorganization and is free to move elsewhere. This process was
described in 1951 by Rosalind Franklin.
NatuRal Pyrolysis of Methane or Ethane
The second, more speculative, method was presented In June 2023 by
John Hanson at a symposium on natural hydrogen at the Geological
Socierty in London titled “Potential to Generate Organic Hydrogen”.
In it, he presents chemical equations that show how methane and
ethane can be converted into hydrogen by natural processes
underground, similar to industrial pyrolysis of methane. The
detailed mechanism is beyond the scope of this article, but John’s
slide presentation can be downloaded
HERE. This is very early work and no doubt revisions and
updates will appear in the literature
.
Possible chemical pathways to organic hydrogen (CAC =
activated carbon)
MANUFACTURED HYDROGEN PRODUCTION
There are over 200 chemical reactions that can produce
hydrogen, some dating back 150 years or so. None could be
considered “Green”. About 48% of commercial bulk hydrogen is
produced by the Steam Reforming Method (SRM), using natural
gas as a feedstock, with CO2
released to the atmosphere, or with carbon capture and
storage (CCS) to mitigate greenhouse gas (GHG) emissions.
Other sources of H2 are from by-products
of the manufacture of ammonia, methanol, and other
industrial chemicals, plus electrolysis of water or
pyrolysis of methane.
In 2012 the natural hydrogen discovery well was tested in
Mali and it has broadened the search for clean geologic
sources.
HYDROGEN PRODUCTION
FROM METHANE USING STEAM REFORMING
The most common method is reacting
water, in the form of super-heated steam (700 – 1100 C), with
methane to form carbon monoxide, which in turn causes the removal of
hydrogen from the methane. The water vapor is then reacted with the
carbon monoxide to oxidize it to carbon dioxide, turning the water
into hydrogen. The process is called Steam Reforming, also known as
the Bosch process. The chemistry is:
1: CH4 + H2O
→ CO + 3 H2
2:
CO + H2O → CO2
+ H2
This reaction is favoured at low pressures but is usually conducted
at high pressures (2.0 MPa). This is because high pressure H2
is the most marketable product, and pressure swing adsorption (PSA)
purification systems work better at higher pressures. The product
mixture is known as "synthesis gas" because it is often used
directly for the production of methanol and related compounds.
HYDROGEN PRODUCTION FROM ELECTROLYSIS OF WATER
When a direct current is run through water, oxygen forms at
the anode (+) while hydrogen forms at the cathode (-).
Typically the cathode is made from platinum or another inert
metal. While this is a proven technology, it supplies only
5% of the World’s demand for hydrogen.
The method presumes that an adequate supply of unallocated
fresh water, (or desalinated sea water or medium depth
oilfield brine) and a source of unallocated electricity can
be found. In many areas, fresh water is already in short
supply and additional draws on surface or near surface water
may be impossible. Deeper sources may also be restricted.
See “Analyzing Water Wells
”
to learn how to locate potential underground sources of
water.
The chemistry for electrolysis is pretty simple:
3: 2 H2O + electricity → 2 H2
+ O2 + heat
Theoretical efficiency (electricity used vs. energetic value of
hydrogen produced) is between 88 to 94% with no impurities in the
water, much less if desalinization is needed. Energy costs of
compression, storage, and transportation to market are also not
included.
HYDROGEN PRODUCTION FROM METHANE PYROLYSIS
Natural gas (methane) pyrolysis is a one-step process that produces
no greenhouse gases. Developing volume production using this method
is the key to enabling faster carbon reduction by using hydrogen in
industrial processes, fuel cell electric heavy truck transportation,
and in gas turbine electric power generation.
Pyrolysis is achieved by having methane (CH4)
bubbled up through a molten metal catalyst containing dissolved
nickel at 1,070 C. This causes the methane to break down into
hydrogen gas and solid carbon, with no other by-products (except
those from maintaining the reactor at the high temperature
required).
The chemistry is deceptively simple, but implementation is tricky.
4: CH4 + heat + catalyst
→ C + 2 H2
The industrial-quality solid carbon may be sold as manufacturing
feedstock or permanently landfilled, it is not released into the
atmosphere and there is no ground water pollution in the landfill.
Methane pyrolysis is in development and considered suitable for
commercial bulk hydrogen production, assuming low-cost methane is
available as both feedstock and heat source. Further research
continues in several laboratories and at least one pilot project.
NATIVE HYDROGEN
FROM SERPENTINIZATION REACTIONS
The hydrogen in the Mali example may have come from a deep
source from mantle degassing, a moderate depth source from
rock crushing in faults, or a shallow source from chemical
serpentinization.
Serpentinization is a form of low temperature metamorphism driven
largely by hydration and oxidation of olivine and pyroxene, creating
serpentine minerals brucite, and magnetite. Under the unusual
chemical conditions accompanying serpentinization, water is the
oxidizing agent, and is itself reduced to hydrogen. This leads to
further reactions that produce rare iron group native element
minerals, such as awaruite and native iron, methane, and other
hydrocarbon compounds, and hydrogen sulphide.
During serpentinization, large amounts of water are absorbed into
the rock perhaps during intense rainy seasons, increasing the
volume, reducing the density and destroying the original rock
structure. The density changes from 3.3 to 2.5 gm/cc with a
concurrent volume increase on the order of 30 to 40%. The reaction
is highly exothermic and rock temperatures can be raised by about
260°C, providing an energy source for the formation of
non-volcanic hydrothermal vents.
Hydrogen is produced during the process of serpentinization. In this
process, water protons (H+) are reduced by ferrous (Fe2+)
ions provided by fayalite (Fe2SiO4).
The reaction forms magnetite (Fe3O4),
quartz (SiO2), and hydrogen (H2).
5: 3 Fe2SiO4 + 2
H2O → 2 Fe3O4
+ 3 SiO2 + 3 H2 +
heat
fayalite + water → magnetite + quartz +
hydrogen
Laboratory studies of serpentinization at high temperature and
pressure show how methane could be produced, lending some credence
to deep-seated gas and oil generation and migration.
6: 18 Mg2SiO4
+ 6 Fe2SiO4
+ 26 H2O +
CO2 →
12 Mg3Si2O5(OH)4
+4 Fe3O4
+ CH4
forsterite + fayalite + water +
carbon dioxide → serpentine + magnetite +
methane
My grade 9 chemistry class didn’t get much past 2H2
+ O2 → 2 H2O,
but equation 6 looks OK to me.
Ocean seeps show both hydrogen and methane emissions. We just have
to find them on land, complete with a hydrogen accumulation, as in
the Mali example. There are more than 100 published reports of
natural hydrogen seeps on land in a dozen countries, treated as
curiosities across many years. Maybe they will lead to a new
industry, just as the oil seeps of antiquity did. (Reference:
Wikipedia)
ACKNOWLEDGEMENT
Thanks to Denis Briere of Chapman Petroleum Engineering Ltd for
contributing information and suggestions for this article, including
illustrations in Figures 3, 4, and 5.
REFERENCES
1. On generating a geological model for hydrogen gas in the
southern Taoudeni
Megabasin, Bourakebougou area, Mali” ACS Letters, 12 June 2016
Denis Briere and Tomasz Jerzykiewicz,
https://doi.org/10.1190/ice2016-6312821.1
2. Hydrogen and Hydronium, Chem-Libre, 2022
https://chem.libretexts.org/Bookshelves/General_Chemistry/Book%3A_ChemPRIME_(Moore_et_al.)/11%3A_Reactions_in_Aqueous_Solutions/11.05%3A_Hydrogen_and_Hydroxide_Ions
3. Hydrogen and Hydronium Technical Data, Production Methods, Serpentinization
Various Wikipedia pages
|