RESISTIVITY IMAGE LOGS
In
1986, the "ultimate" dipmeter was developed by
Schlumberger, called
the formation microscanner (FMS). Later versions were called
formation micro-imager (FMI). There are now similar services
available from other suppliers. The tool is generically known as
a resistivity image log.
Using an additional 27 electrodes
on each of two dipmeter pads of the dipmeter; each pad records 27 microresistivity
curves spaced 1/10 inch apart on the borehole surface. Each pad
covers a 2.8 inch wide portion of the circumference of the well
bore. Several passes over the interval will often provide virtually
complete coverage of the rock face.
The
resistivity traces are translated into images based on their relative
resistivity values, in either black and white or colour. The
resistivity data provided by this tool are of very high resolution,
in the order of a few millimeters. Thus, a substantially large
data array must be handled, and it is an obvious challenge to
process and display this information in a way which facilitates
its interpretation. This is resolved through a point to point
mapping of the resistivity traces into a spatial image, each
pixel in the image display having a gray scale or colour value
associated with a particular resistivity level. Subsequent
interpretation benefits from the close relationship between this
image and core photography.
The gray
scale or colour spectrum can be stretched or squeezed in the computer
to enhance certain features, such as porosity, fractures, or shale
laminations. Images can be plotted at the same scale as the core
photographs for comparison.
It is traditional to show low resistivity (shales and open
fractures) as black, shading to white for high resistivity
(tight streaks and healed fractures). Porous sands and shaly
sands will be grey or a mix of yellow-brown with darker colour
suggesting higher resistivity and possibly lower porosity. In
rare cases, such as tar sands and oil shale, the colours may be
reversed to make hydrocarbon layers black and shales lighter
colours.
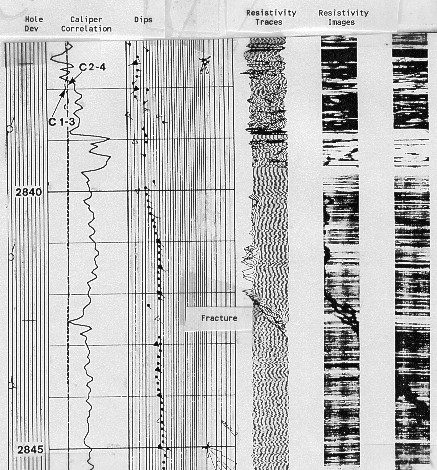
Resistivity image log showing calculates dips, raw curves, and
image log from a two pad FMS device.
A
resistivity image logging tool with fewer (sixteen) electrodes per pad, but
with four or eight imaging pads, is now available, and provides
better coverage of the wellbore wall than the two pad version.
The electrodes are smaller, allowing for higher vertical resolution, but
are spaced to provide the same wall face coverage, about 2.5 inches
per pad. In an 8 inch diameter hole, electrode coverage is about
80% and in a 6 inch hole is greater than 100%. This overcomes
one of the major complaints about the FMS, namely the number of
passes needed to obtain a complete image of the well bore. An
example is shown below.
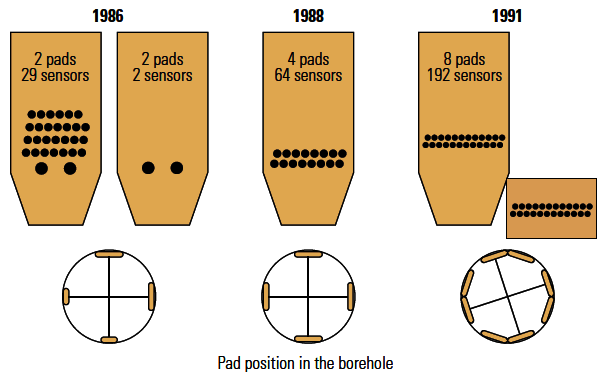
Evolution of the Schlumberger resistivity image
log pad design, starting with the original two pad FMS (located on 2
of the 4 pads of the SHDT dipmeter), the four pad FMI (which could
be twinned with another tool at 45 degree offset for better borehole
coverage), and the eight pad FMI-HD tool (which gives about 98%
coverage in an 8" boreho;e).
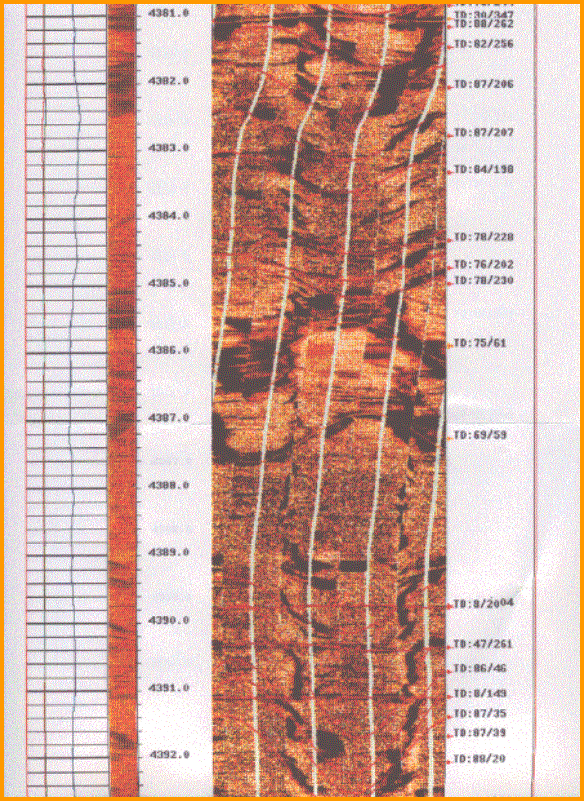
Four pad FMI log in fractured granite reservoir showing
computed dip angle and direction. North is at centerline of
image.
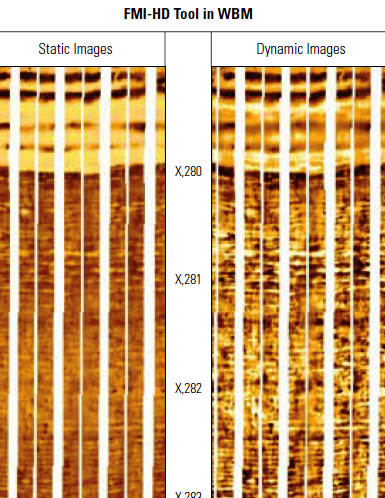
An 8-pad FMI-HD log in a gas shale. Left track is static (fixed
colour scale) image. Right track is dynamic image, in which a
running average type of colour scaling is used to amplify
resistivity contrasts, making easier to see stratigraphic features,
faults, and fractures.
The
primary use of the tool is definition of bedding plane dips, for identification of irregular features,
such as vugs and fractures, for accurate sand counts in thin bedded
zones, and for identifying stratigraphic features. If sufficient
rock face is imaged, dips can be found by digitizing the bedding
planes visible on the microscanner image, or by automatic computation
using all valid image traces.
The dips found by FMI dip processing
are superior to CSB or LOC dips because a larger number of resistivity
traces can be used in the calculation. They can be computed automatically
and displayed on the FMS image. In addition, calculated dips can
be edited or removed, and new bed boundary correlations picked
with a mouse on an interactive CRT image. Thus dips that pass
or fail preconceived processing criteria can be deleted or added
as the analyst desires. Dip tadpoles can be coded by colour to
indicate bedding, cross bedding, fractures, or faults.
RESISTIVITY IMAGE LOG EXAMPLES
Images
courtesy of Schlumberger
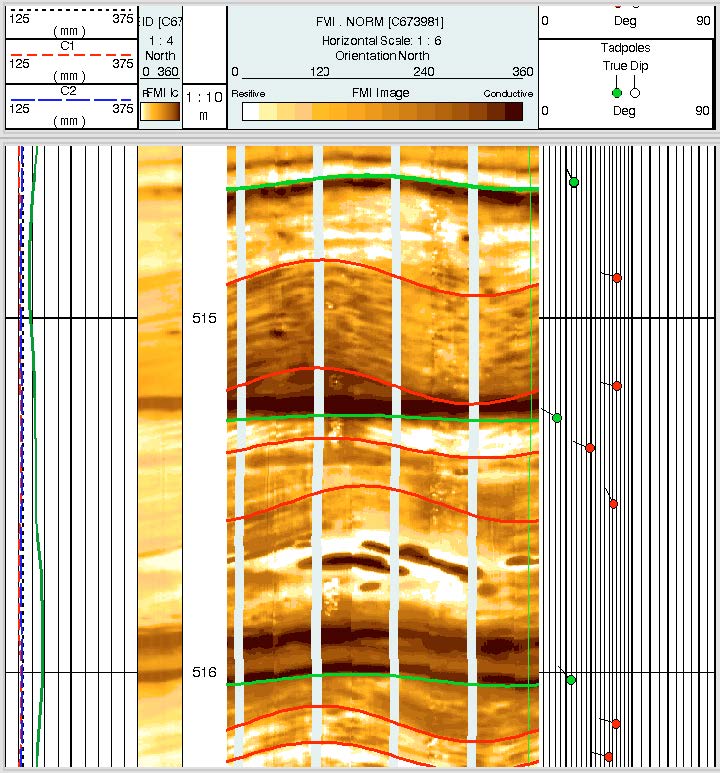
Left track is caliper, right track is formation dips. Dip in
the cross beds indicate paleocurrents to the WNW direction. This
is confirmed by the imbricated shale clasts.
Channel orientation is ESE to WNW
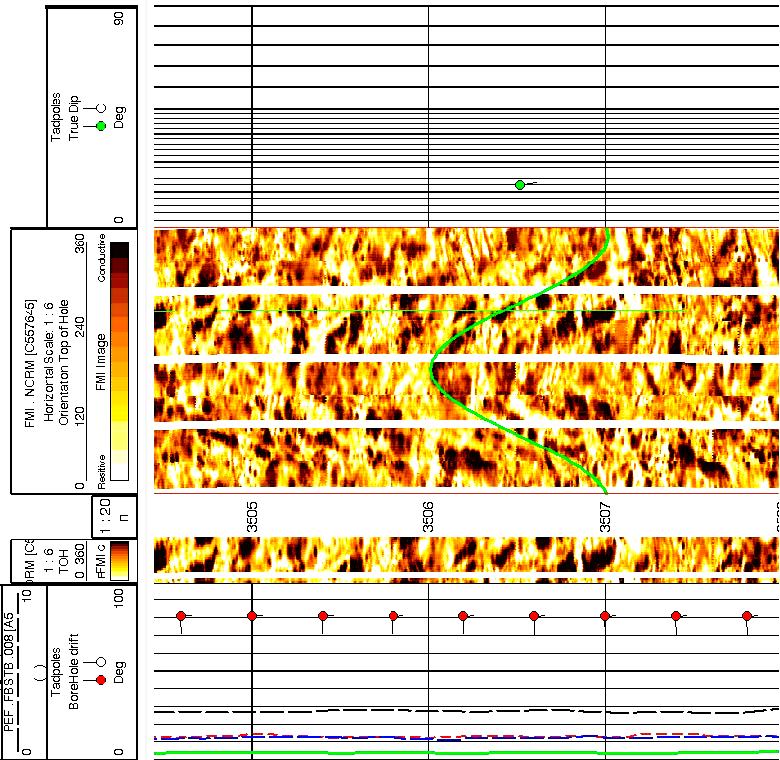
Resistivity images are best dislayed horizontally for
horizontal wells-- toe of the well is on the right. Top track is
formation or fracture dips, bottom track is borehole deviation.
Images illustrate fenestral porosity within a carbonate unit
intersected by a horizontal well. Actual well productivity with
this type of reservoir is higher than convention open hole logs
would indicate.
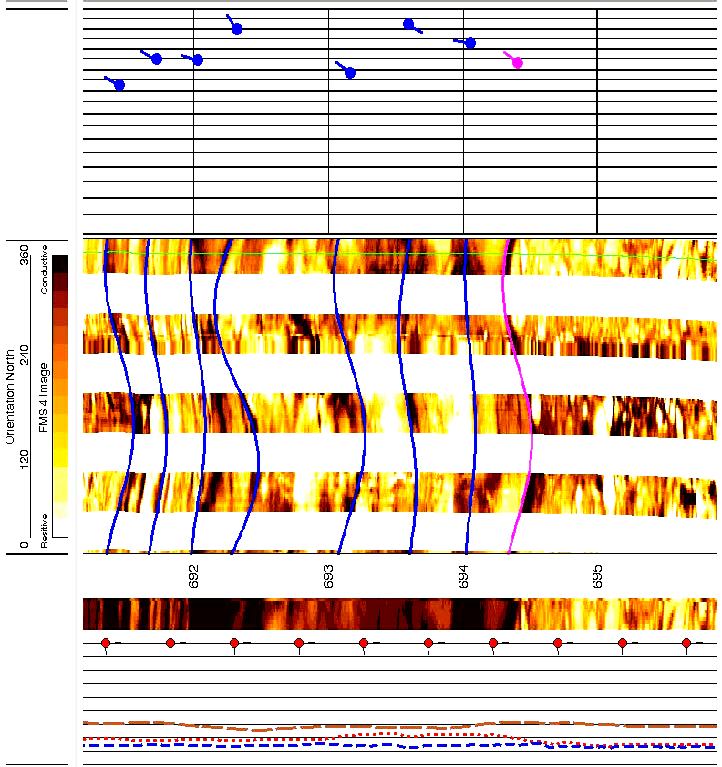
The slimhole FMS* provides imaging capabilities in boreholes
as small a 114mm (4.5 in). This Image reveals a normal fault and
fractures in a horizontal well. Fault block downthrown to the
N45E direction with 63' dip.
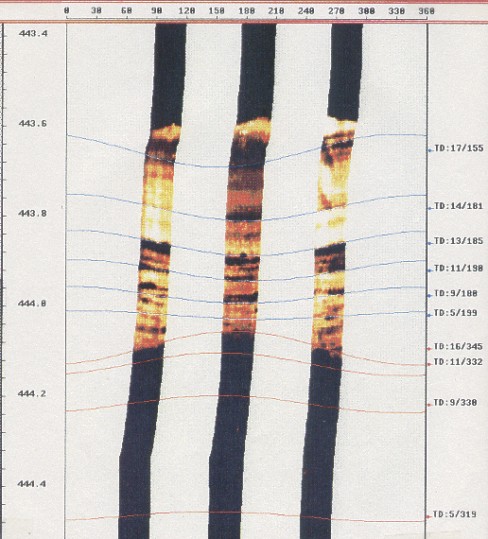
Expanded colour scale image of tight streak (light
brown) on resistivity image shows detail of porosity laminations
- light colour is tight, darker colours are more porous, black is
shale.
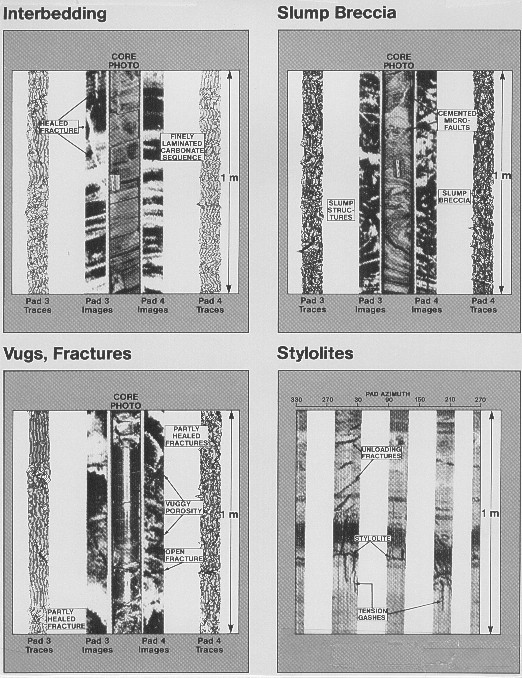
Formation microscanner images in various environments
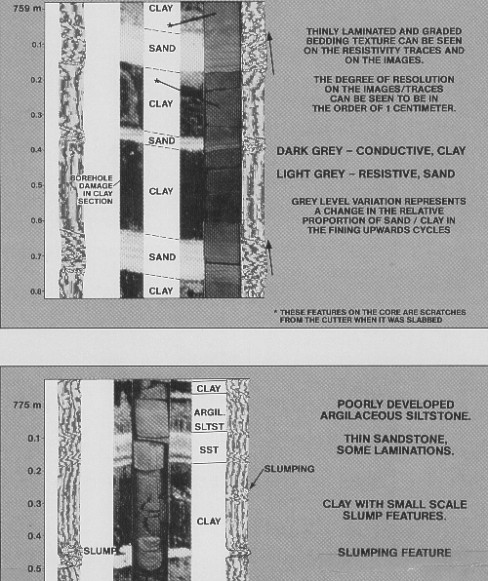
Formation microscanner images in various environments
RESISTIVITY IMAGE LOGS IN OIL-BASE MUD (OBM) SYSTEMS
Material in this section is based on "Imaging - Getting the Picture
Downhole" edited by Tony Smithson, Schlumberger Oilfield Review,
Sept 2015.
Conventional resistivity image logs require a conductive mud system
to operate. In oil based (nonconductive) mud, a knife blade
electrode, or "scratcher pad", version of the resistivity image log
was available from several suppliers in the later 1980's. The
scratcher systems worked reasonably well for bedding dips and sand
counts, but could not see fractures very well, because they filled
with nonconductive mud.
Schlumberger
introduced a new typw of oil-based mud imaging log (OBMI) for use in nonconductive
mud systems in 1988. It uses micro induction resistivity
measurements instead of the usual electrical resistivity pads. It
had 4 pads with 2 rows of 4 electrodes on each pad, operating in the
10 to 20 kiloHertz range. A second tool could be added at 45 degree
offset to increase borehole coverage. Resolution vertically and
horizontally was about 1 cm, much coarser than the conventional
tools (FMS, FMI). It was not very effective in fractures and there
were other artifacts that caused shadow events, making the image
difficult to interpret in some cases.
The micro-induction technique of
older OBM imagers and scratchers of even older dipmeters have been
replace by Schlumberger's Quanta Geo tool. It makes a
capacitive measurement at two frequencies in the megaHertz range
(instead of the kiloHertz range used in conventional tools) and then
derives the best image from the optimal frequency. The tool has 8
pads so the coverage is good compared to other OBM tools. The
electrodes are surrounded by guard electrodes that focus current
through the mud and mudcale into the formation.
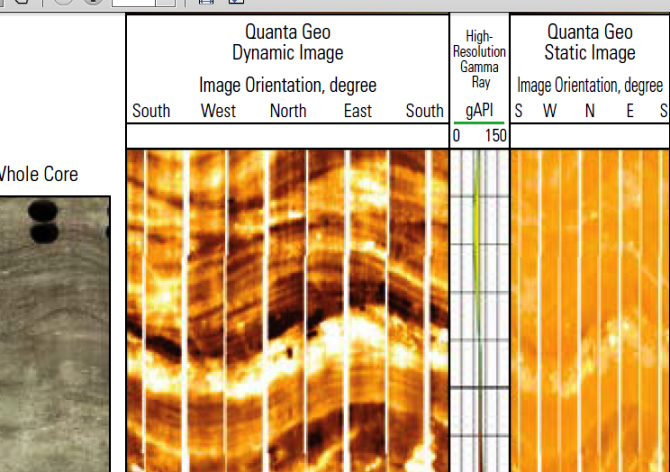
Core image (left), static and dynamic images from Quanta Geo
image log
The capacitive measurement allows
the processing to determine the amplitude of the current / voltage
ratio and the phase shift between the two measurements. The ratio
gives the electrical impedance of the rocks. At the frequencies
used, the impedance is not directly proportional to resistivity, but
the images produced have the same general appearance as conventional
image logs. Vertical resolution is 6 mm and horizontal resolution is
3 mm, comparable to the FNI-HD in water based mud. Both open and
healed fractures are quite evident on the Quanta Geo output as high
resistivity (white) sinusoids. An acoustic image log uld be used to
distinguish open from healed.
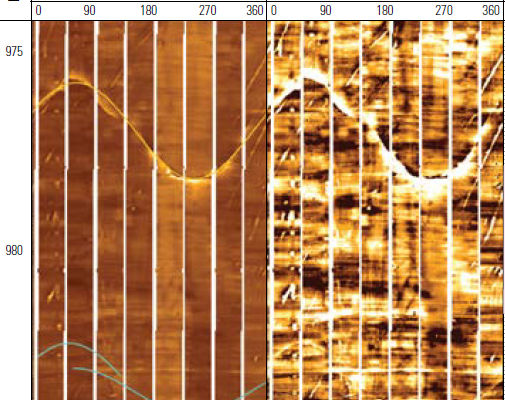
Fractures (white sinusoids) in oil base mud using Quanta Geo image
log. Open fractures cannot be distinguished from healed as both are
resistive in OBM.
LOGGING
WHILE DRILLING IMAGE LOGS
Measuring
resistivity at the drill bit is part of a normal logging while
drilling operation. Three resistivity curves sampling different
depths of investigation are sent uuole in real-time, along with any
other logs in the tool string. Since the tools are rotating while
drilling, the deep resistivity scans the entire wellbore as the tool
moves slowly down hole. The scanned data is too much to send uphole
in real-time and is recorded, then recovered at the surface
when the drill string is tripped for a bit change. Some newer RAB
tools can transmit sufficient dats to create an image uphole for
near real-time display. Once all the image data is assembled, it can
be processed to obtain dip indoemation i a manor similar to wirekine
image logs.
Since the density log
is also a directionally focused tool that rotates, it too can give
an image, although with less contrast than resistivity.
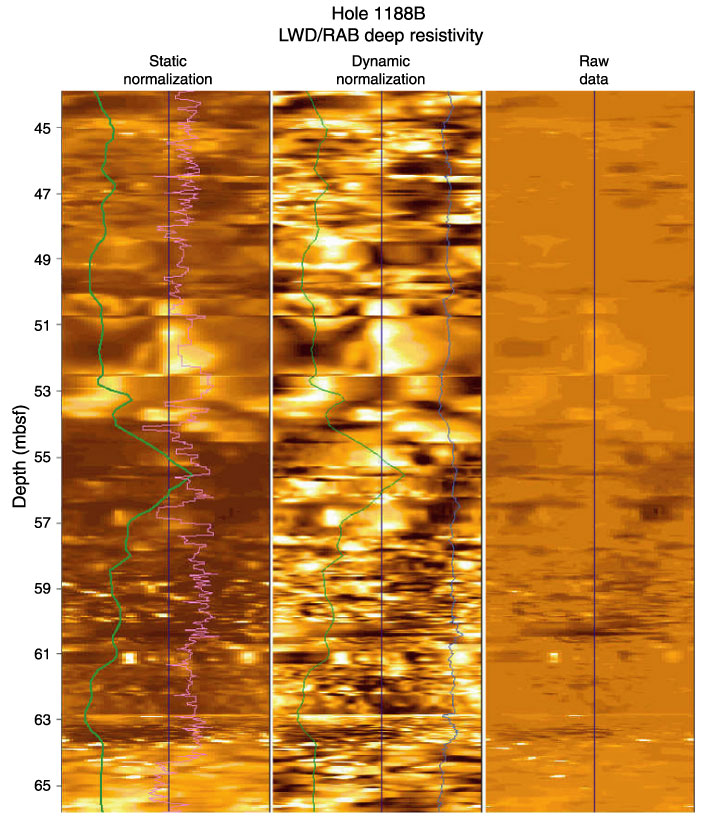
Deep resistivity image from a logging while drilling tool called
Resistivity at Bit (RAB). As for all image logs, black represents
low resistivity, white is high resistivity. North is at centerline
of each image. Since this is a deep resistivity, light colours could
mean hydrocarbons or low porosity. Comparison to an open hole FMI or
an LWD density image log could resolve the ambiguity.
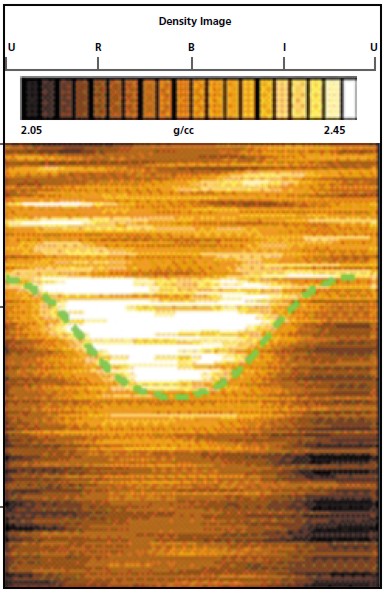
LWD density image log, black is low density (shale or porous),
white is high density (tight). Comparison to RAB
can identify
hydrocarbons: light colour on RAB + dark colour on density image =
hydrocarbon. This image is from a
horizontal well; bottom side of
hole is at the centerline of the image.
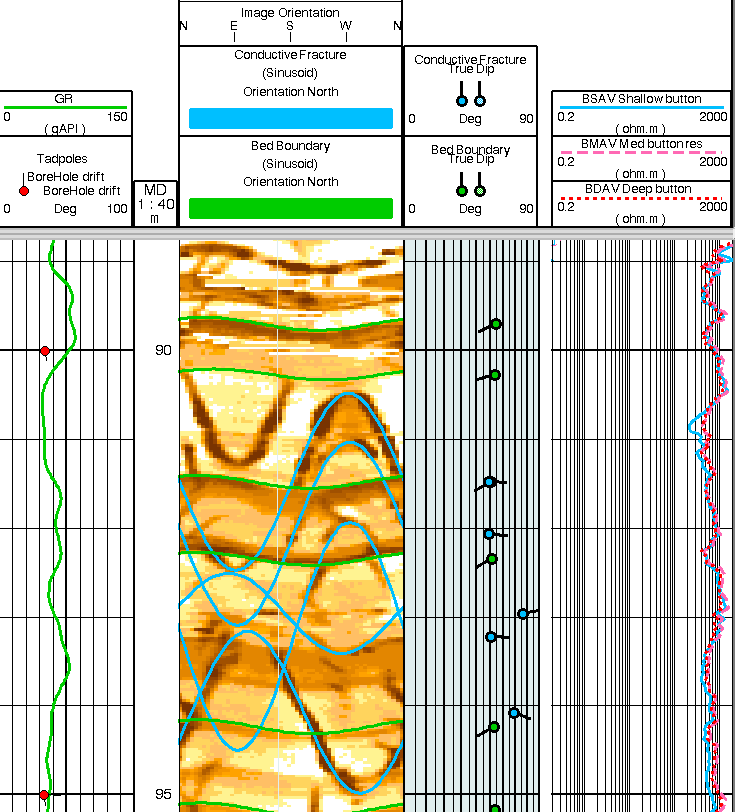
Typical Resistivity-At-Bit (RAB) image log shows gamma ray at
left, resistivity image, dip tadpoles, and 3 resistivity curves
on the right. This image illustrate open fractures (with blue
traces) cross-cutting bedding (in green).
IMAGE LOGS FROM DIPMETERS
Dipmeters and image logs are an essential for assessing structure
and stratigraphy of reservoir rocks and for Identification of
fracture intensity and fracture porosity. Tool design has improved
considerably since its introduction in 1986.
A
resistivity image log has about 10 times the spatial resolution of an
acoustic image log
and 500 times the amplitude resolution, due to the difference
in contrast between the resistivity and acoustic impedance ranges
measured by the respective tools.
The
standard tool works only in conductive mud in open hole, and a
specialized tool is available for non-conductive mud. It does not
work in cased holes.
An extension of the
stratigraphic high resolution dipmeter (SHDT) processing provides a core-like image
of the borehole, using the LOC dip correlations and the measured
resistivity curves. The program is called STRATIM (Schlumberger
trademark). This image predates the resistivity image log by a
few years.
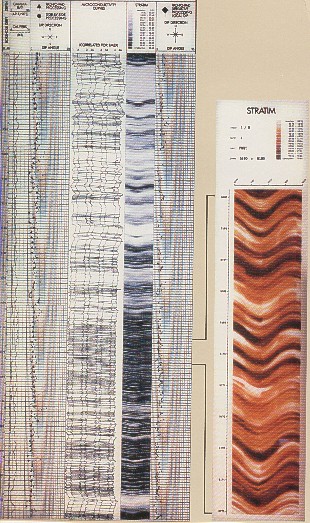
An
example is given on the left. The program produces a 360 degree
image of the borehole wall by interpolating between the eight
resistivity measurements from the eight electrodes on the SHDT
pads. Images can be coded in gray scale or colour. Dark gray or
dark colour usually represents conductive, often tight shale,
beds and light colour resistive, often porous sand, beds. If shales
are more resistive than sands (or carbonates), the colour scheme
can be reversed to keep shales looking dark.
The dipmeter curves are rotated to their true azimuth but are
not adjusted to true dip. The dips seen on the image are as they
would appear on the surface of a conventional core. The trace
of a plane dipping bed forms a sinusoidal curve when the image
of the borehole wall is unwrapped and laid flat, as they are in
these images. Bed boundaries, dipping beds, slump features, and
fractures are easily seen, if present. Images can be enhanced
as in Formation Microscanner processing, but processing is cheaper
because much less data is manipulated.
A
similar program, called DIPVUE is available from Western Atlas,
illustrated below. Here the 3-D image can be rotated in real
time to view the artificial "core" from any direction. In addition, most core service companies
can provide core photographs and dip logs from core data for comparison
with log derived borehole images.
The
colour convention is to show low resistivity in black and high
resistivity in white (or yellow). This makes shale beds black and
clean sands white, with shades of grey or brown representing shaly
sands. In carbonates, the same rules are used, but white may now
mean tight streaks with grey representing porosity. The colour scale
can be stretched and squeezed to enhance the image for a particular
situation.
Note
that a planar, dipping, bedding plane will trace a sine wave on a
circumferential image, such as those shown above
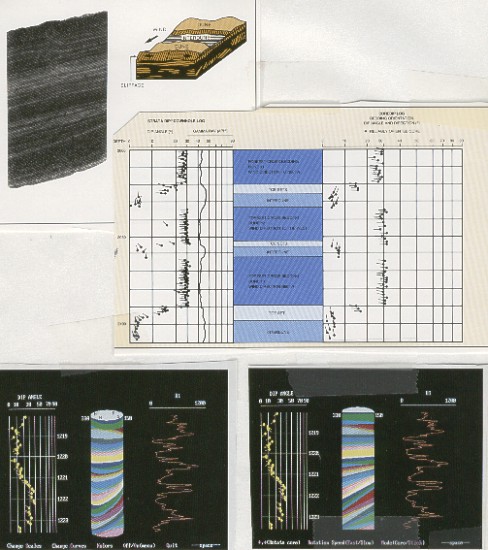
DIPVUE image created from dipmeter data
RESISTIVITY IMAGES FROM
MODERN LATEROLOGS
Azimuthal resistivity image logs
(a form of laterolog) and high resolution laterologs can be
displayed as images as well as resistivity curves.
Below is a sample of an array induction (AIT) log and an azimuthal
resistivity (AIR) log, the latter showing the azimuthal image log
presentation.
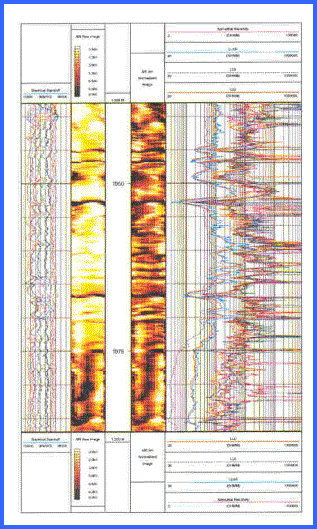
Comparison of array induction log (left) and azimuthal resistivity laterolog (right). Curve complement and
presentations vary considerable with age and contractor. The image
log on the azimuthal resistivity presentation is "poor man's"
resistivity microscanner log, giving a reasonable sand count
regional dip, and some fracture information. A real microscanner
image is shown for comparison (left image).
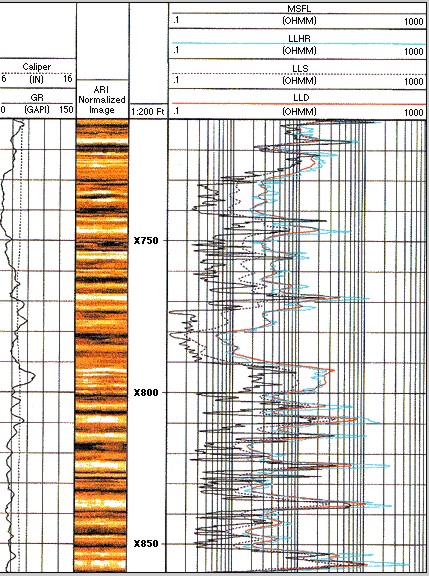
High resolution laterolog showing deep invasion and high
resolution image.
All curves are focused to
about 8 inches. This tool is not azimuthal so image shows flat-lying
beds even when dip is present.fs
|